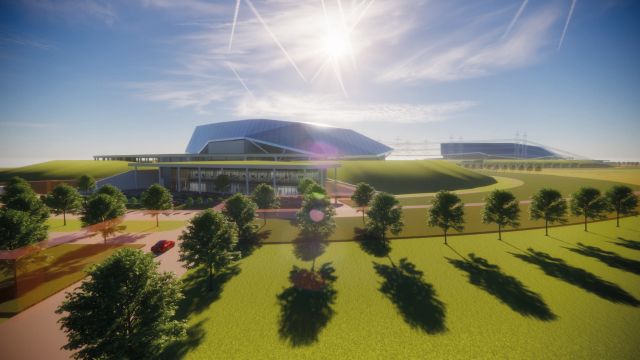
Small-scale nuclear power plants: What's behind SMR concepts?
SMR definition
According to the definition commonly used today, the abbreviation SMR stands for Small Modular Reactor. In this context, “small” usually refers to an electrical output of up to 300 megawatts (MWe). Reactors with an output of approx. 1 to 10 MWe are also referred to as microreactors. The modular character of the plants is expressed by the fact that the essential components of a primary circuit - i.e. in particular the actual reactor vessel and the subsequent cooling circuit - are all contained in a single module. In addition to transportability, the idea behind this is to prefabricate the essential parts of a power plant in a factory with such a module and thus minimise the work on the construction site. Individual modules with low output should then be able to be linked together to form a larger power plant if required. In the long run, standardised serial production should reduce manufacturing costs, licensing risks, and construction times.
In the past, a different definition was used by experts. The International Atomic Energy Agency (IAEA) used the term “Small and Medium Sized Reactors”. Reactors with an output of up to 300 MWe were described as “small” and those with an output of between 300 and 700 MWe as “medium”. Nowadays, however, the former definition of SMRs as small modular reactors is used almost exclusively. The classification of plants with an electrical output between the upper limit of 300 MWe (according to the more recent definition) and conventional nuclear power plants, to which the IAEA counts those with an electrical output of approx. 700 MWe upwards, is therefore not clear.
Overview of SMR concepts
According to the OECD's Nuclear Energy Agency (NEA), there are currently almost 100 concepts for reactors worldwide that according to current interpretations are classified as SMRs. A few of these have already been built or are under construction, while the majority are in various stages of design or more concrete planning. According to the NEA, 35 of the concepts are not currently being actively pursued.
Most of the concepts that are comparatively close to being realised or have already been built are so-called light water reactors - the technology used in the vast majority of conventional nuclear power plants.
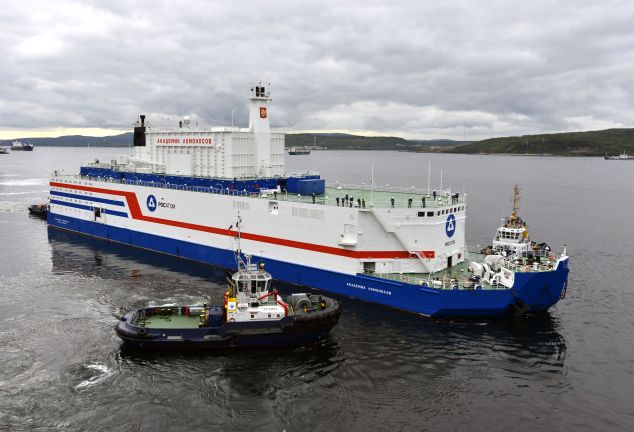
Examples of these SMR concepts include the Akademik Lomonosov floating nuclear power plant, which has been supplying the remote port city of Pevek in Siberia and neighbouring mines with electricity and heat since May 2020, and CAREM (Argentina), which is currently under construction.
Small light water reactors, some of which have been used for decades in icebreakers, submarines or on aircraft carriers, are also sometimes listed as SMRs. In terms of electrical output alone, however, the majority of heavy-water-cooled reactors in India (200 MWe) or the four reactors operated at the Russian Bilibino nuclear power plant (12 MWe) could also be categorised as SMRs; the boundary to reactors such as the Soviet VVER-440 (440 MWe) or Qinshan-1 (330 MWe) in China would be almost fluid with this understanding.
In addition to these light water SMR concepts, numerous other concepts are based on reactor technologies that utilise coolants and/or moderators other than water and that differ significantly from light water reactors in terms of their reactor physics. The reasons for this are manifold: In some cases, other technologies are intended to achieve a safety gain compared to light water reactors; in a number of concepts, other applications such as the extraction of process steam or process heat for industrial applications, the use of transmutation to reduce radioactive waste, the breeding of fuel or the burning of weapons-grade fissile material are to be made possible in addition to or instead of electricity generation.
Corresponding concepts are sometimes also referred to as Advanced Modular Reactors, i.e. “advanced” or “novel” reactors, and are categorised as the so-called “fourth generation” of reactors. However, a number of the SMR concepts categorised in this way are based on technologies that were conceived decades ago and in some cases also realised in prototype reactors, albeit without reaching market maturity or becoming more widespread. Examples here include high-temperature and breeder reactors, which were also built in Germany in the form of the THTR-300 at Hamm and the SNR-300 at Kalkar.
In its Advanced Reactor Information System, the IAEA categorises new reactor concepts, including SMRs, in terms of the coolant and moderator used. However, this categorisation does not do justice to all the special features of SMRs or new reactor concepts as a whole - developments and special features of thorium or high-temperature reactors, for example, are not taken into account. Nevertheless, a categorisation based on the coolant is particularly useful (see drop-down menu).
- Light-water-moderated and cooled SMR concepts: This type of SMR has probably been serving longest and used most widely. A differentiation is made here between boiling water reactors and pessurised water reactors.
- Heavy-water-moderated and cooled SMR concepts: So-called heavy water contains the hydrogen isotope deuterium instead of normal hydrogen atoms and therefore absorbs fewer neutrons than normal water. This means that more moderated neutrons are available for nuclear fission. Instead of enriched uranium, natural uranium could therefore also be used as fuel in heavy-water-moderated SMRs. The CANDU-like Indian reactors can be cited as an example here. The use of heavy water in SMRs also potentially allows the utilisation of thorium, from which fissile uranium is then bred during operation.
- Gas-cooled SMR concepts: Gas-cooled SMR concepts rely on the use of gases, such as helium or carbon dioxide, as coolants. Compared to other SMR types, they reach significantly higher coolant temperatures (up to 1,000 degrees Celsius (°C)) and it should therefore be possible to use them to generate process heat in the chemical or petrochemical industry. Low-temperature processes, for example for district heating utilisation, could be connected downstream with these concepts. Two gas-cooled high-temperature reactor modules with a capacity of 250 MWe each have been in operation at the Chinese Shidaowan nuclear power plant since December 2023.
- Liquid-metal-cooled SMR concepts: In SMR concepts with liquid metal cooling, lead, lead-bismuth or sodium should be used as coolants. The metals are characterised by a high boiling temperature and a high thermal capacity. Uranium in combination with plutonium or other transuranium elements will be used as fuel. In order to prevent contaminated primary coolant from reacting with the water-steam cycle, these concepts usually provide for a component cooling system. The core outlet temperatures should be around 750 °C. Steam and heat could then be extracted in a temperature range of 500 to 700 °C for other purposes. A lead-cooled SMR, the BREST-300, is currently being built in Russia. Commissioning is planned for 2026.
- Molten-salt-cooled SMR concepts: Finally, other SMR concepts envisage molten salts both as a coolant and as a fuel carrier. It is assumed that the molten salts tested to date are stable up to temperatures of 1,400 °C. The heat transport properties of molten salts should allow the reactors to be built with significantly smaller dimensions compared to gas-cooled reactors with the same output. The high operating temperatures should allow high efficiencies and heat extraction for industrial high-temperature processes. An experimental thorium molten-salt reactor is currently being operated in China. According to media reports, this will be followed by the construction of a commercial 10 MWe reactor by the end of this decade.
Realisation status
Including the plants listed in the overview, only five SMRs are currently in operation or under construction (not including small reactors in nuclear submarines, aircraft carriers or icebreakers). Whether SMRs will be built on a significantly larger scale in the future is difficult to predict at present.
In addition to the technical and regulatory challenges that exist in particular for SMR concepts that are not based on light water reactor technology, economic considerations also currently tend to speak against the widespread use of SMRs. One of the reasons for this is that the electricity generation costs in a large nuclear power plant are lower than in a smaller plant. It also plays a role here that the hoped-for cost reductions through serial production could only be achieved with higher quantities, which in turn would also require regulatory standardisation. Insufficient economic viability has e.g. recently led to a new build project planned by the manufacturer NuScale in the USA being cancelled at the end of 2023. In spring 2024, NUWARD, a subsidiary of the French utility EdF, also cancelled its SMR concept, which had been developed over four years, following discussions with potential customers as the expected generation costs were unlikely to be achieved with the concept for technical reasons.
In contrast, however, it should also be noted that the development and subsequent use of SMRs is strongly supported both politically and financially in a number of countries. This applies to countries such as the USA, Russia and China, which are home to a significant proportion of the developing companies and rely heavily on nuclear energy for their own power supply. In Europe, besides France, the United Kingdom should be mentioned in particular which with its recently founded Great British Nuclear state agency is running a competitive process for SMR developers with a view to the subsequent state-subsidised construction of SMRs and recently tendered over one billion pounds sterling in funding in this context. In some Central and Eastern European countries such as Poland, Romania and the Czech Republic, plans for the use of SMRs are also being driven forward; in some cases, specific sites have already been selected and regulatory reviews have been initiated. Finally, the European Commission has also launched an initiative with the European Industrial Alliance on Small Modular Reactors, which aims to accelerate the development and deployment of SMRs in Europe.
What must also be taken into account is that from the perspective of potential users, certain possible applications of a low-carbon power supply through SMRs could potentially outweigh economic disadvantages. For example, the announcements by Amazon and Google in October 2024 to invest in the development and construction of SMRs to ensure a stable and climate-friendly power supply for their data centres made the headlines.
In addition, SMRs are to be used for so-called repowering in countries such as the USA and Poland - this involves continuing to utilise the sites of old coal-fired power plants with their grid connection by replacing them with SMRs of comparable capacity. Finally, in countries such as Russia and Canada, the focus is also on supplying remote regions or industrial sites that have no or only an inadequate connection to the national grid.
Novel SMRs and safety
Developers believe that new SMR concepts have safety advantages over large nuclear power plants. Among other things, passive safety functions, some of which are already used in conventional nuclear power plants, are cited as being decisive for this. These systems do not require electrical energy for activation and operation but are driven by e.g. gravity. In certain SMRs, passive safety systems are intended to enable automatic shutdown without the need for an external power supply or human intervention. Cooling should also be possible passively by gravity, convection or evaporation and thus without the use of electrically driven pumps to circulate the coolant. However, the latter variety would in turn require a certain design height.
By using alternative coolants and passive safety systems, some SMR concepts can rule out certain accident scenarios that have to be taken into account in conventional nuclear power plants. For example, studies suggest that a core meltdown can be ruled out for the Chinese HTR-PM. The damage potential of an assumed serious accident in an SMR is also reduced compared to a large nuclear power plant in that SMRs are to be equipped with significantly smaller quantities of nuclear fuel - the amount of radioactive substances that could be released into the environment in the event of an accident would be correspondingly lower.
However, depending on the concept, these potential advantages are offset by new safety challenges. In the concept of a sodium-cooled SMR, for example, it must be ensured that the metallic sodium will not come into contact with oxygen, as it ignites easily. In addition, the highly corrosive effect of molten salt places special demands on the properties of the materials used to manufacture e.g. the coolant lines. Finally, innovative concepts must also take into account the very limited operating experience compared to classic light water reactors. Many of the safety improvements that have been developed for light water reactors are based on the evaluation of events. Corresponding empirical values are naturally not available for many of the new SMR concepts.
Work of GRS on SMRs
As part of its research and its activities as authorised expert organisation, GRS has been looking at the safety of SMR concepts at a conceptual level. In a study funded by the Federal Economics Ministry, for example, the need for research into the further development of existing simulation programs for assessing the safety of nuclear power plants was examined.
An assessment of the safety of SMRs is only possible to a limited extent for various reasons. It is true that statements can be made as to whether a particular SMR concept is plausible in terms of its safety-related design and complies with recognised principles. However, it is not possible to make reliable statements on the basis of concepts alone as to whether a plant would be capable of being licensed in accordance with the current state of the art in science and technology or the applicable regulations.
Such assessments require a great deal of information that cannot be obtained from a concept alone or is not yet available. This primarily includes the specific technical realisation of the concept, for example the exact technical specifications of the safety-relevant components and the materials used. However, the safety of a nuclear installation also depends on the characteristics of the specific site, for example with regard to seismic activity or possible flooding.